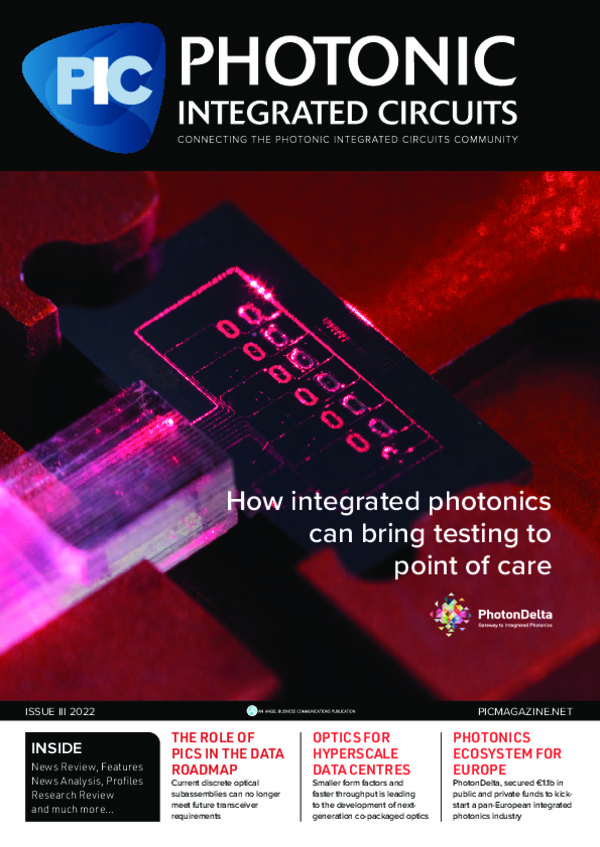
The Role of PICs in the Data Communications Roadmap
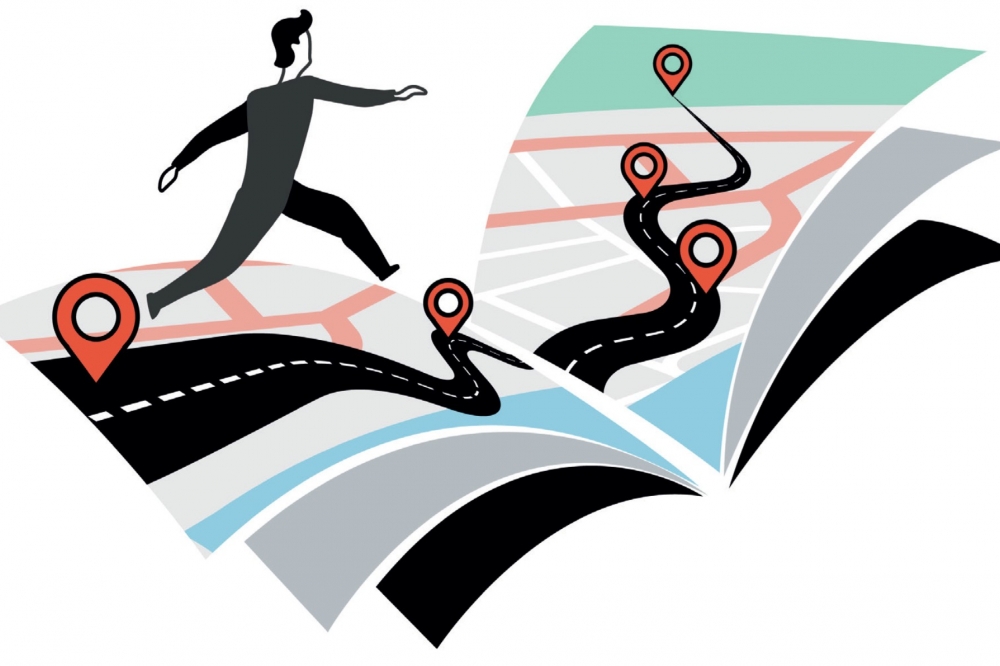
As bandwidth consumption continues its meteoric rise, the complexity of optical links and the transceivers within them continue to increase in complexity. Yet at the same time, data center operators are demanding more efficiency. Current discrete optical subassemblies can no longer meet future transceiver requirements. The time has come for PICs to deliver on their promise and save the future of optical transceivers. In this article, the role of PICs in future optical transceivers is examined.
By Jim Theodoras, HG Genuine
A quick peruse of the leading optical industry news sources reveals that Photonic Integrated Circuits (PICs) continue to be an area of substantial hype and investment. Given the global impact of silicon integrated circuits, it is no surprise that the optical industry has spent decades trying to replicate the success. PIC categories, types, and technologies are as widely varied and numerous as the color swatches at a paint store. A more recent area of focus is the use of PICs in optical communications, as they hold the promise of breaking the bandwidth logjam that is rapidly building up with traditional techniques.
Bandwidth consumption is outpacing the pace at which the optical communication industry can respond. Three ways of increasing an optical channel’s throughput is to simply go faster (increase bits per second), go wider (increase the number channels), or get smarter (more complicated modulations than just on/off). The industry has done an admirable job of increasing laser modulation speeds over the decades, but more recently speeds cannot keep up with needs. The industry recently made the difficult jump to PAM4 modulation, which doubled throughputs, helping with the inability of speed to scale fast enough. But even faster lasers and higher order modulations have not been enough, and so the number of channels in each link continues to climb. Looking at standard Ethernet, the physical link definitions have grown from one to four to eight lanes, with 16 lanes on the horizon.
As Datacom has struggled to keep pace with bandwidth demand, Ethernet links have not only become faster, but wider as well
The aforementioned bandwidth logjam is created by conflicting market river currents. While the complexity and number of channels of optical communication links increases, the end customers who are buying and hence funding this growth are demanding greater efficiency in power, price, and space. For example, one popular 800G optical transceiver is a 2x400G-FR4 in OSFP and QSFP-DD form factors. This product contains two sets of 4 colors of 100G, each channel having a laser, modulator, collimation lens, and a shared wavelength multiplexer on the transmit side alone. Producing an optical transceiver with this high of a component count is certainly doable, but it is doubtful continuing down this path will yield the longer term efficiency gains end customers are looking for. The excitement, work, and investment in PICs is an effort by the industry to break through this barrier.
So if PICs are the answer, what should a PIC look like for use in an optical communications link, and more specifically a pluggable transceiver? Optical transceivers consist of a wide range of mechanical, electrical, and optical componentry. These parts need to be grouped together by the most appropriate technology. For example, the host PCB can contain the paddle card connector, microcontroller, power supplies, memory, and serve as a mounting point for the metal clamshell housing. The key problem is what functions should a PIC replace, and the answer depends on the PIC technology being used. In traditional optical transceivers, the optical subassemblies are referred to as the Transmitter Optical Subassembly (TOSA) and the Receiver Optical Subassembly (ROSA), or sometimes combined as a TROSA. Generally speaking, the goal of PICs is to replace the TOSA, ROSA, or both in the TROSA.
One common mistake is to estimate a market value of a PIC replacement on the total costs of the subcomponents that it is replacing. For example, if a PIC replaces a laser, modulator and wavelength multiplexer, then the PIC’s market value is the sum of the laser+modulator+mux cost. While this seems reasonable, the logic is flawed, in that the true market value of a PIC could be higher or lower than the sum of the costs. In order to understand why, the economic lifecycle of transceivers must be considered.
The ideal optical transceiver would consist of just a DSP and PIC. This 4xFR4 example shows 4:1 color laser sharing enabled by Photonic integration
The economic lifecycle of an optical transceiver is complex. Here production volume is plotted against cost of manufacturing. Note there are several distinct phases where pricing is determined by differing factors.
Given this new perspective, PICs destined for optical transceivers must be evaluated in a new light. As aforementioned, PIC technologies and designs vary widely. Each option’s value added must be carefully weighed against the value subtracted. Examples of value-added features include but are not limited to: No laser alignment, isolator, or Thermoelectric cooler (TEC) needed. Also, fewer voltages and control loops might be needed. Examples of value-subtracting features of a PIC might include too many AC coupling capacitors, special drivers, special voltages, or bias-T’s needed. An ideal PIC would be able to use the built-in drivers inside the latest DSPs, include integrated colored lasers, modulators, wavelength multiplexer/ demultiplexer, need no isolator, have integrated photodiodes and transimpedance amplifiers, and be compatible with the same solder reflow process as the DSP package. At the time of this writing, no PIC technology yet exists that delivers all of these functions, but several promising technologies are very clo
Assuming the perfect PIC did exist, what might a transceiver look like? A popular optical transceiver variant used in Hyperscale data centers today is known as a FR4, which uses 4 color channels at 100Gb/s each to deliver 400Gb/s aggregate bandwidth up to 2km. To fully leverage the density advantage of a PIC, 4 of these FR4 could be shoehorned into single transceiver, thus maximizing the sharing of not only common functions, but potentially 4:1 laser sharing as well. Ideally, the DSP and PIC would be the only major integrated elements, and both could be flip-chipped onto a single substrate. That electrical/optical substrate would then be mounted on the PCB that would provide the paddlecard edge connector, power supplies and microcontroller.The 4xFR4 example illustrates the potential of PICs in optical transceivers. Making something smaller does not necessarily make it lower power, and often has the opposite effect. However, the greater level of integration of PICs allows for common resource sharing, improving power per bit. Also, making something smaller does not necessarily make it cheaper either, and it often has the opposite effect. Again, the greater level of integration of PICs allows for fewer manufacturing steps in what is a much more complicated optical assembly. All the benefits of optical integration parallels those offered by electronic integration, which is why the optical communication industry is pursuing PICs with such fervour.