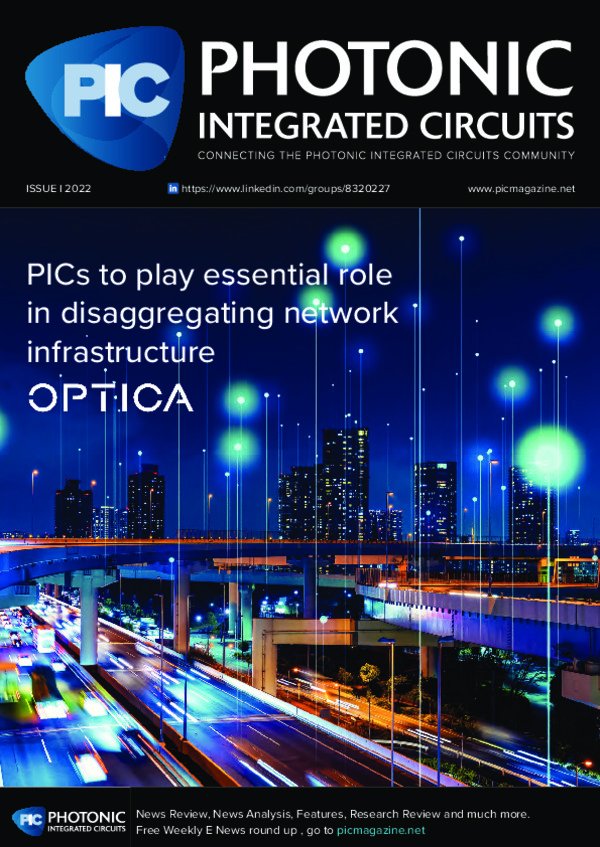
Hybrid photonic integration for sensing and communications
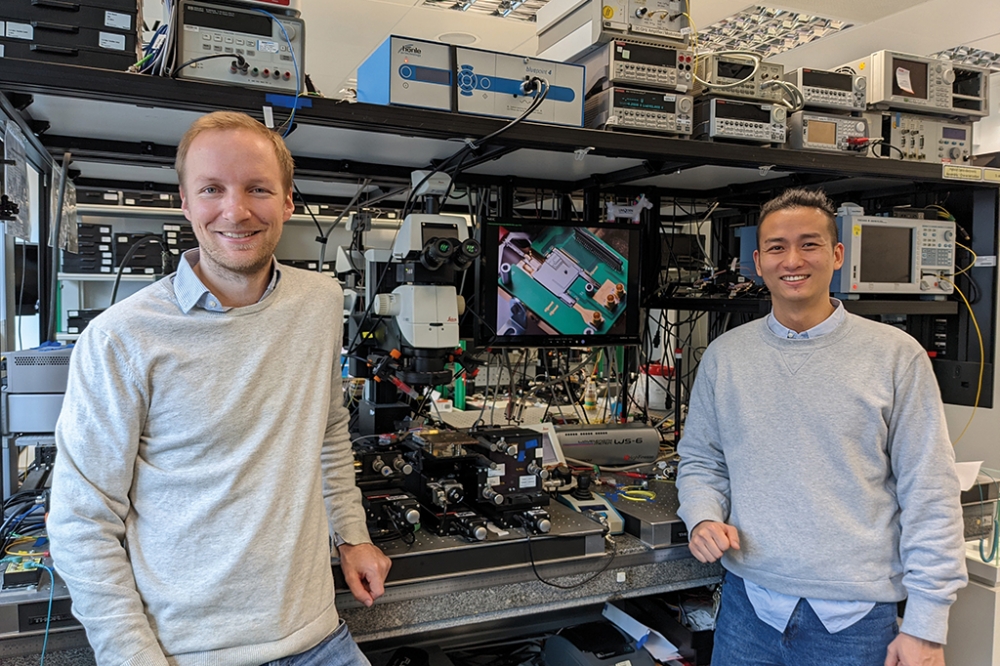
In 2018 Fraunhofer HHI first described PolyBoard in the pages of PIC
Magazine. PolyBoard is the Institute’s polymer-based, highly flexible
hybrid photonic integration platform designed to support numerous
applications. PolyBoard offers a straightforward integration approach
through its micro-optical bench. In this article, Fraunhofer researchers
describe key innovations since the platform’s introduction and ways
that continuing work is enabling photonic integration beyond telecom and
datacom.
BY TIANWEN QIAN, HAUKE CONRADI AND MARTIN SCHELL, FRAUNHOFER HHI
IN LATE 2018, the PIC magazine featured the PolyBoard, a polymer-based hybrid photonic integration platform developed at Fraunhofer HHI. This article described the first steps and concepts towards integrating optical isolators, circulators, and nonlinear crystals with the micro-optical bench. Moreover, ultra-widely tunable lasers and the possibilities of 3D photonic integration with vertical interconnects were introduced. Three years later, numerous advances within the further development of the PolyBoard were made.
This paper describes the progress made in the PolyBoard-implementation of integrated optical isolators and nonlinear crystals. Utilizing the wide transparency range of polymers, all functionalities of the PolyBoard can also be shifted towards lower wavelengths, even down into the visible. The second chapter of this article reviews tunable lasers at 1064 nm and 785 nm for applications beyond Telecom and Datacom.
Furthermore, complex photonic integrated circuits (PICs) for THz generation and detection highlight the versatility of hybrid photonic integration. As an essential step towards commercialization of these PICs, this article concludes with automated hybrid photonic assembly.
Fig 1: (a) DBR laser with integrated isolator. The DBR laser consists
of an indium phosphide (InP) gain element, phase shifter (PS) and
Bragg-grating. The output of the micro-optical bench is coupled directly
to a single-mode fiber (SMF). (b) Demonstrated tuning of the laser
across a bandwidth of 17 nm. Different colors correspond to different
tuning currents of the Bragg grating. The laser is operated under 10%
back reflection.
The micro-optical bench
For optical isolators to work, they have to break the Lorentz reciprocity. In free-space optics, this is most often achieved with magneto-optic materials but the implementation of such materials in PICs is a challenging field of research. Especially when it comes to the integration with additional other optical components, for example lasers.
At OFC 2021, HHI presented the first tunable distributed bragg-reflector (DBR) laser with an integrated optical isolator based on the micro-optical bench [1]. The micro-optical bench is an on-chip free-space section consisting of two graded-index (GRIN) lenses coupled to polymer embedded waveguides with the help of precisely etched U-grooves. The first GRIN lens enlarges the beam diameter of the input waveguide and creates a collimated beam with low beam divergence. After the free-space section, the second GRIN lens couples the light back into the waveguide. This enables the low-loss integration of bulk elements, like free-space isolators, into the PolyBoard. The DBR laser protected by the isolator comprises an active indium phosphide gain section coupled to a polymer chip with a waveguide-inscribed tunable Bragg grating and a phase shifter. The fabricated device is depicted in Figure 1.
Fig 2: (a) Polyboard-integrated periodically poled lithium niobate
(ppLN) crystal. (b) Spectrum of second-, third, and fourth-harmonic
generation (SHG, THG, FHG), pumpt at 1550 nm.
A maximum output power of 5.6 mW and a tunability of 17 nm was measured for this device. The excess loss added by the integrated isolator is only 1 dB, while it provides an optical isolation of 38 dB across the whole tuning range of the laser. The functionality of the PIC was tested during a 10 Gbit/s transmission across a 10 km fiber link with 10% of the laser power coupled back onto the chip as optical feedback. Even at these high levels of optical feedback, the isolator ensured a stable operation of the laser, while a coherence collapse appeared in a reference laser without optical isolator.
Besides optical isolators, the micro-optical bench enables the integration of any other materials. In [2], the integration of periodically poled nonlinear lithium niobate crystals for second-harmonic generation (SHG) was demonstrated by pumping it with a femtosecond pulse laser at 1550 nm, resulting in a conversion efficiency of 8 %. Figure 2 depicts the PIC used to create SHG together with the optical spectrum. Due to higher-order phase matching, not only the second but also the third and fourth harmonic at 517 nm and 388 nm are observable.
Besides SHG, nonlinear crystals are also useful to create photon pairs or entangled photons via spontaneous parametric down-conversion, targeting applications in quantum communications like quantum key distribution. Furthermore, the integration of nitrogen-vacancy-rich diamonds enables the development of sensitive magnetic field sensors. Both these applications are currently under investigation. Overall, the micro-optical bench is a key enabler for transferring quantum and non quantum lab experiments into photonic chips.
Tunable lasers at 1064 nm and 785 nm
Many applications beyond telecommunication require wavelengths well below 1300 nm. This is why we extended our hybrid laser concept to shorter wavelengths. By replacing the indium phosphide (InP) with gallium arsenide (GaAs) gain material, combined with the efficient thermo-optically tunable gratings on the PolyBoard platform, tunable laser designs at shorter wavelengths are also possible [3].
Figure 3: The packaged laser diode in a butterfly housing with PolyBoard based hybrid tunable laser in the middle.
Figure 3 shows an assembled laser with coupled GaAs gain element built up in a butterfly housing. The measured grating tuning spectra for wavelengths at 1064 nm and 785 nm are shown in Figure 4, 10 nm and 8 nm tuning range are achieved respectivly. The measured output power for both lasers is 2 mW and 1 mW respectively, with linewidths <200 kHz. The magneto-optic material used for the optical isolator described earlier also works at 1064 nm and 785 nm. Thus if required, the co-integration with an optical isolator is also possible at shorter wavelengths.
Figure 4: Hybrid tunable laser lasing at (a) 1064 nm with 10 nm tuning range and (b) 785 nm with 8 nm tuning range.
Using the same concept, tunable lasers targeting visible light wavelengths are currently being developed in the framework of the PolyChrome project. Together with other available building blocks like thin-film filters (TFFs) for polarization control and spectral filtering, this provides a photonic integration platform that can target numerous applications in quantum technologies, medical or life science and spectroscopy.
Figure 5: Packaged hybrid Polymer THz transmitter (left) and THz
receiver (right) PICs comprise of indium phosphide (InP) SOA, phase
modulator, photodiode, photoconductive antenna (PCA), Si3N4 TriPleX PIC
and PolyBoard in the middle. The corresponding functional schematics are
shown below. The concepts of on-chip optical frequency comb generation
and injection locking, as well as the photonic THz transmission via InP
photodiode or the photonic THz detection via photoconductive antenna are
realized on these PICs.
Complex hybrid photonic integration: THz transmitters and receivers
The versatile functionalities offered by PolyBoard also attract the integration with other material platforms, which makes complex hybrid PICs that combine numerous photonic chips possible. The concept of such complex hybrid integration using PolyBoard was recently demonstrated in the framework of EU Project TERAWAY [4].
Figure 5 depicts a hybrid THz transmitter and receiver comprising an InP semiconductor optical amplifier (SOA), phase modulator, photodiode, photoconductive antenna (PCA), Si3N4 TriPleX PIC and the PolyBoard in the middle. Taking the THz receiver as an example (Figure 5, right): a tunable laser followed by two cascaded phase modulators generates an optical frequency comb (OFC), which is used as a master signal for frequency-stabilization of two other tunable lasers by means of injection locking.
These two stabilized laser signals mix at the photoconductive antenna (PCA), enabling the photonic detection of the upcoming wireless THz signal covering 92 GHz to 322 GHz. The PolyBoard itself provides the functionalities such as tunable lasers, thermo-optically adjustable couplers and optical isolators [5, 6]. The hybrid approach enables using the adequate material for each function in order to maximize the performance of the whole PIC.
Towards automatic assembly
All PICs in this article require several assembly steps, ranging from lens- and crystal-alignment to coupling gain elements or even modulators. An essential step towards commercialization of these PICs is the automation of all assembly and alignment processes. In close collaboration with ficonTEC, a versatile ficonTEC assembly machine is in use to demonstrate these automatic processes.
Figure 6: ficonTEC assembly machine with customized tools for fiber and gain chip gripping, TFF pick up and insertion.
As depicted in Figure 6, this machine has customized tools for holding fibers and GRIN lenses, gripping gain chips, picking up thin-film filters (TFFs) and inserting them into the PolyBoard. These pickup tools provide the handling of all components required in the assembly, while top- and bottom-view cameras together with dedicated image recognition algorithms, optical feedback loops and optical axes with submicrometer movement precision enable the fully automated chip-assembly.
The TERAWAY receiver during characterization. Hauke Conradi (left) and
Tianwen Qian (right) are research associates at Fraunhofer HHI.
Conclusion
In this paper, we gave a brief overview about our work on tunable lasers at different wavelengths, the possibility to integrate isolators or even nonlinear crystals and complex hybrid PICs for THz transmission. All these developments result in a highly versatile photonic integration platform that enables PICs that target not only classical communications, but also THz communications or quantum communications, e.g. entangled photon sources for quantum key distribution, and sensing applications.
Further reading
References
[1] H. Conradi et al., “Tunable DBR Laser with Integrated Optical Isolator,” 2021 Optical Fiber Communications Conference and Exhibition (OFC), 2021.
[2] H. Conradi et al., “Second Harmonic Generation in Polymer Photonic Integrated Circuits,” J. Lightwave Technol., vol. 39, no. 7, pp. 2123–2129, 2021, doi: 10.1109/JLT.2020.3046371.
[3] M. Kleinert et al., “Hybrid Polymer Integration for Communications, Sensing and Quantum Technologies from the Visible to the Infrared,” in 2021 European Conference on Optical Communication (ECOC), Bordeaux, France, Sep. 2021 - Sep. 2021, pp. 1–4.
[4] T. Qian et al., “Hybrid Polymer THz Receiver PIC with Waveguide Integrated Photoconductive Antenna: Concept and 1st Characterization Results,” 2022 Optical Fiber Communications Conference and Exhibition (OFC), 2022.
[5] D. de Felipe et al., “Polymer-Based External Cavity Lasers: Tuning Efficiency, Reliability, and Polarization Diversity,” IEEE Photon. Technol. Lett., vol. 26, no. 14, pp. 1391–1394, 2014, doi: 10.1109/LPT.2014.2324760.
[6] David de Felipe et al., “Recent Developments in Polymer-Based Photonic Components for Disruptive Capacity Upgrade in Data Centers,” J. Lightwave Technol., JLT, vol. 35, no. 4, pp. 683–689, 2017.